- Systems methods in environmental impact assessment
- §4.1 Costs and benefits
- §4.2 Environmental impact assessment
- §4.2.1 Direct effects
- §4.2.2 Indirect effects
- §4.2.3 Geographic extent of environmental impact
- §4.2.4 Systems analysis and modeling
- §4.3 Energy and Materials
- Material cycles and material cycling
- Eutrophication
- Trophic levels
- §4.4 Populations
- §4.5 Communities & Ecosystems
- §4.6 Systems analysis and ecology
- §4.6.1 Measurement
- §4.6.2 Monitoring
- §4.6.3 Analysis
- §4.6.4 Description
- §4.7 Impact on the Physical Environment
- §4.8 Environmental Toxicants
- §4.8.1 Environmental Material Cycling
- §4.8.2 Geographic Extent of Impact
- §4.8.3 Effects Over Time
- §4.8.4 Biological Concentration of contaminants
- §4.9 Direct Environmental Impacts
- §4.10 Water Related Impacts
- §4.10.1 Manipulation of Local Hydrology
- §4.10.2 Thermal effluent
- §4.10.4 Material Releases
- §4.10.5 Impacts on Soils
- §4.10.6 Subsurface Geology
- §4.11 Indirect Impacts
- §4.12 Impact on Biological Systems
- §4.13 Assessment of Damage to Biological Systems
- §4.13.1 Commercial and Game Species
- §4.13.2 Species Without Commercial Value
- §4.13.3 Loss of Diversity
- §4.13.4 Disruption of Natural Succession
- §4.14 Effects of Industrial Construction
- §4.15 Effects of Industrial Production
- §4.15.1 Heat
- §4.16 Irreparability of Biological Systems
- §4.16.1 Cost of Repair
- §4.16.2 Rate of Repair
- §4.17 Impact on Air
- §4.18 Problems in Determining Causes of Environmental Damage
- §4.18.1 Space
- §4.18.2 Time
- §4.18.3 Synergism
- §4.19 Social and Economic Effects of Industrial Development
- §4.20 The Metropolitan Suburban Setting
- §4.20.1 Changes in age composition
- §4.20.2 Increase in Income
- §4.20.3 Increased employment
- §4.21 Limitation of Analysis Techniques
- §4.22 Negative Effects
- §4.22.1 Income Inequality
- §4.22.2 “Leakage” of Benefits to Surrounding Areas
Environmental Impact Assessment— costs, benefits, risks
Siting industrial facilities
The year after Warren E. Burger, Chief Justice of the United States Supreme Court lamented, “The painful fact is that the courtrooms of America all too often have “Piper Cub” advocates trying to handle the controls of “Boeing 747″ litigation.” and that he added, “that by no means are all the “Piper Cub” advocates recent law graduates.” He was referring to, among other ill-advised cases cluttering the dockets the spate of cases filed under NEPA, the National Environmental Policy Act of 1970 by ill-prepared newly minted “environmental” lawyers. In 1974, this chapter was written for a textbook on the “Energy Crisis” commissioned by the West Publishing Company after the Arab Oil Boycott of late 1973.
The chapter sought to create a reasonably precise and objective framework for rational discussion of the criteria for and analysis of the effects of site selection for electric power generation facilities. It is appropriate for rational discussion of siting any controversial public works project and evaluate legislation, administrative action, and regulatory proposals which may have adverse but often unsuspected effects on the environment.
§4.1 Costs and Benefits
Cost-benefit analysis as a technique for evaluating the economic desirability of a proposed industrial development project is not new. The concept of assessing the total costs and benefits of projects involving the generation, distribution and use of electricity, such as power plant siting, is not new, and such costs and benefits can be expressed in environmental, social, and economic terms. The task of evaluating costs and benefits involves hard trade-offs among conflicting interests, requiring that statements of costs and benefits must be made explicit. In order for assessment of costs and benefits to be useful, both the magnitude of the cost or benefit and the probability of the cost or benefit must be specified. The probability of cost or benefit is particularly important in evaluating potential costs/ benefits and long term or indirect costs/benefits. Since analysis of costs and benefits is almost always somewhat subjective, the importance of making the methods of evaluation explicit cannot be over-emphasized.
It is the public that must make clear statements of priorities after costs and benefits have been specified. Statements of relative values of public and private goals, and onstraints of the natural environmental system must guide all industrial location decisions.
§4.2 Environmental impact assessment
Environmental impact refers to the accumulated effects of a particular action on the natural, social and economic environment of the region within which such effects can be demonstrated.
In the past, environmental impact assessment generally has considered only effects on the natural environment measured as immediate effects upon existing organisms, or levels of undersirable materials added to the air and water. In considering facilities for the generating and distribution of electrical energy, such as power plants and transmission lines, it is important to assess environmental, impact by measuring, estimating and predicting the total direct and indirect effects on natural and human ecological systems both on and off the site over short and long time-spans. The task of measuring and estimating indirect effects is neither easy nor inexpensive, but the long-term benefits to society should justify the costs. There is a pressing need to examine effects on the socio-economic environ ment concurrently with studies of effects on the natural environment since these systems strongly interact. The effects of industry location on social and economic systems must be considered not only in terms internal to those systems such as employment and income, but also in terms external to such systems particularly effects on the natural environment which accompany the location of a new industry.
Three areas of particular concern to citizens considering the environmental impact of industrial development are:
- the criteria used in evaluating the environmental impact of industrial development,
- the information upon which the criteria are based, and
- the procedures by which decisions are made and implemented.
The natural and socio-economic environment of any region ultimately rests in the custody of some governmental organization which responds to that collection of numerous and varied interests commonly lumped together as the “public.” Decisions as to whether an industrial plant will be built and if so, where it will be built must be based on information concerning the effects of the decision on natural en vironmental systems and the socio-economic system of a region. The tradition of making industrial location decisions primarily on the basis of industrial economics and the immediate economic considerations of a political unit is changing under the National Environmental Policy Act (NEPA) and more specifically under similar state legislation. Benefit to the industry may often be obtained only at great expense to natural systems and the postulated benefits to any political unit must be carefully examined. Both direct and indirect effects of the plant siting must be analyzed over time.
Among the most urgent needs of those assessing the impact of industrial siting is the need for meaningful information. An important difficulty with present infor mation on the socio-economic systems and natural environmental is its inaccessability to the public. Data scattered among a number of public agencies, or buried in technical reports which are available only to a very limited number of decision makers and generally only consider narrow areas of interest.
Concerned citizens should insist upon advance public disclosure of siting alternatives and energy use options, and sufficient lead time to review all the data listed in this section before full committment to any energy generation, distribution and utilization option or site selection is made.
The geographic boundaries within natural systems-watersheds, air sheds, biological communities, physiographic and geologic formations, and soil patterns are seldom if ever coterminous with political boundaries. Watersheds, for example, may be contained within a single township or may be of international extent. Many important watersheds appear to be at least of multi-county size and some of those are multi-state in dimension. Citizens in any political subdivision which may be affected by a particular land use or energy policy decision should participate in the decision-making process.
§4.2.1 Direct effects
Probable direct effects on the socio-economic system of the region can be determined quickly from precise descriptions of the proposed plant (payroll, public facilities required, total value of plant, etc.). Probable direct effects on the natural environment can also be estimated from studies of the pro posed site when the plant size, construction methods, production processes, resources required, and materials to be added to the natural system are known.
§4.2.2. Indirect effects.
Effects on natural systems and socio-economic systems may occur over long time periods and wide geographic areas. Application of on going analyses of the systems involving the proposed site is the necessary means of determining the probable long term and spatially distributed effects. Studies assessing the significance of general system dynamics, materials cycling, synergism and economic multiplier effects must precede industrial location decisions. If well defined models for predicting the behavior of systems are available, they may be the only substantial scientific framework to consider impacts of long term duration and wide geographic extent in both natural and socio-economic systems.
§4.2.3. Geographic extent of environmental impact
The geographic extent of the impact of industrial site selection on the natural and socio-economic systems of a region is a critical element of industrial site decision-making. The indirect social, economic and natural environmental effects of siting almost any major industrial facility extend beyond the municipality, township, or county in which the site is located. Clearly, decisions based on assessment of total environmental impact must be made by all governments whose area of geographic concern includes the area of significant environmental impact from the project.
Determination of the magnitude and extent of specific effects of industrial plant siting in a particular location still requires site-specific data collection and analysis. Collection of data on the natural environment necessary to determine the probable extent of environmental impact attributable to a particular industrial plant requires a minimum of two years in most of the continental United States.
Collection of social and economic data and analysis of the social and economic composition of a region is important in the determination of socio-economic impact, however such studies can usually be completed in a shorter time period.
§4.2.4. Systems analysis and modeling
Considerable emphasis in this Chapter is placed on the need to understand the system of interacting elements or component parts of the social, economic, and natural environments because the most important indirect effects of generating, distributing and consuming electrical energy cannot be determined without a description of the behavior of the interacting elements in the system. For example, the detrimental effects of mercury released into the water system as industrial waste were not understood until systems analysis showed that the mercury was transformed biologically into an organic mercury compound which was then dangerously concentrated in the food chain. Similarly the total effect of the siting of a particular industry in a region cannot be determined until socio-economic analysis reveals the probable associated development which will accompany the new plant siting.
Since systems analysis includes a description in measurable terms of the behavior of a system over time it makes it possible to predict changes in the system over time and space.
One of the most important uses of systems analysis is in determining which data are most significant in determining the environmental impact of energy generation, distribution and utilization. Decisions regarding which data should be included in a comprehensive case study can be made more intelligently within the context of the systems analysis of a conceptual model.
§4.3 Energy and Materials
.
In order to consider the effects of environmental contamination certain basic ecological concepts must be considered, and while biological communities, for the most part, are inordinately complex, there do seem to be certain real and distinct levels on which biological communities are organized and on which they can be studied.
The study of living organisms can be organized on a series of different levels corresponding to the different ways in which they are studied. Organisms can be studied at the molecular level, or at the level at which molecules are organized into cells, or at the level where cells are organized into tissues and organs, or at the level of the entire individual organism. The study of ecological systems deals with the higher and much more loosely organized levels where individuals are organized into populations, populations into trophic levels, and trophic levels into ecosystems.
Two general processes impose a gross patterning and organization upon ecological systems: energy flow and material cycling.
Living systems depend on the flow of energy from the sun, and over any appreciably long period of time, subject to the Laws of Thermodynamics, the amount of energy entering a biological system upon the earth is roughly equal to the amount of energy lost as heat to outer space. The existence of both source and sink is essential since the earth must maintain this energy balance .
Some incident solar radiation is trapped by plants. The energy is then transfer red from one part of the biological system to another, each transfer involving biochemical reactions during which thermal energy is liberated, so that eventually all of the energy first trapped by photosynthesizing plants leaves the system in the form of heat.
The earth is thus an open system with respect to energy, however, with respect to matter, the earth is essentially a closed system, the amount of matter being generally fixed, with merely the state changing. Energy flows throughout the system and in so doing establishes material cycles. The water cycle is an example.
Material cycles and cycling
For some chemical elements the natural cycle is fairly simple. For others, including many of the elements used by biological systems, the cycle is more complex. Man now uses almost all of the elements thereby complicating and disrupting natural material cycles.
The most complete cycles tend to be those in which there is a gaseous phase such as the hydrologic (water) cycle, the oxygen-carbon dioxide cycle, and the nitrogen cycle.
These cycles tend to be balanced and the amount of material in any one phase, such as oxygen in the atmosphere, tends to remain relatively constant. Small disturbances are corrected naturally and changes are usually only temporary. However, these cycles may not be able to return to the steady state following disturbances sufficiently large to cause severe disruption of the cycle.
Sedimentary cycles involve movement of materials from land to sea and back again. Elements moving through such cycles tend to take thousands or even millions of years to cycle, and the cycles tend to be less complete than those with a gaseous phase. In the sedimentary cycles materials are leached or eroded from rocks on land and carried by rivers to the oceans. Most of the material is deposited on the shallow inshore areas of the oceans and eventually returned to the land when these sediments are raised into mountains by the geological process of uplifting. There is some slight loss of certain elements such as calcium and phosphorus since small amounts of these elements are deposited in deep ocean sediments. Such losses in the geochemical cycle are probably permanent, at least as far as the time frame of mankind is concerned.
Human beings can use the enormous energy sources available to them to interfere in a massive way with the cycling of materials. In particular, by mining and drilling operations elements are removed from more or less concentrated forms and dispersed, a process which clearly cannot persist indefinitely for any element. This process of dispersion represents a substantial disruption of the cycling process. Copper, for example, once mined and dispersed as pipe and wire, will not recycle naturally to an extent again useful to human populations. Of course, we could recycle it artificially within our industrial system.
The exploitation of naturally occurring elements by the human species leads to pollution. Pollution may be defined as the interference or perturbation of the natural cyclic processes so that materials accumulate where they shouldn’t. With respect to some elements this results, in part, from the simple fact that because we extract materials from the earth at very high rates, the natural cyclic processes cannot cope with the increased rate at which these materials are returned to the system.
Eutrophication
Possibly the most widely discussed example of this breakdown in natural cyclic processes is eutrophication. In eutrophication, the rate of input is too high and degradation continues in the absence of oxygen by different organisms, than those which normally degrade waste using the oxygen dissolved in the water. The results are usually offensive to man. This change in the quantity of input results in a change in the quality of the biological system, illustrating the point that biological systems frequently respond to changes in the rates of input of materials or energy in a non-linear fashion, and often manifest threshold level effects. (See Chapter 5.)
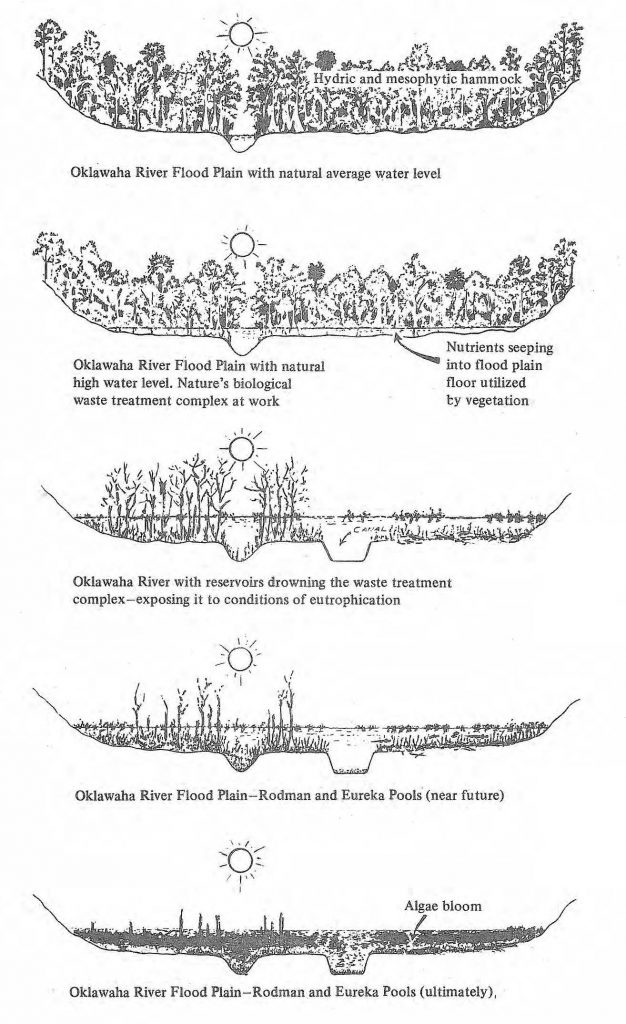
Ocklawaha Flood Plain
Trophic levels
Energy flow and material cycling can be considered in greater detail by examining trophic levels.
In the oceans, energy is trapped mainly by phytoplankton, which provide the food for very small invertebrates called zooplankton, which in turn are eaten by larger invertebrates and small fish, which are attacked and eaten by larger fish and other animals, which in turn become the food or prey of still larger animals and man. Each link in this food chain may be treated as a trophic level-a group of organisms feeding on similar food sources. Typically the organisms in any trophic level are larger than those in lower trophic levels. Food chains, or more realistically, food webs, provide the pathways along which energy and nutrients move throughout an ecosystem, and the trophic levels represent the transfer points along the way at which nutrients are utilized and energy exchanged.
At any particular point in time, in any particular ecosystem, there is no doubt that trophic levels may exist in reality; however, many organisms, regularly, or at different stages of their life cycles are found in different trophic levels. Trophic levels are, in ecology, an abstraction, however useful in conceptualizing the structure of ecosystems and investigating the flow of energy and material throughout the system.
For any biological system to persist there has to be a basic trophic level which traps solar energy and fixes it in a form consumable by the organisms in higher trophic· levels. This function is performed by green plants and this first level is generally referred to as the producer level.
Just as necessary for the completion of nutrient material cycles are organisms which can break down the complex organic molecules of plant tissues. Ultimately this function is performed by micro-organisms (bacteria) and fungi.
Intermediate activities involving the green plants of any ecosystem include those trophic levels in which the plants are consumed while alive or upon death but prior to decomposition by micro-organisms.
There are two general series of trophic levels commonly encountered in an ecosystem . One, the herbivore chain, has plants as the basic trophic level, herbivores which eat green plants, predators which eat other animals, particularly herbivores and parasites. The other series, the detritus or decomposer chain, has dead materials as the basic trophic level. The distinction between the two food chains involved is not complete, since snails, for example, eat both living and dead organisms.
Plants, the primary producers, synthesize organic molecules from inorganic molecules in the environment utilizing solar energy in the process. This energy is fixed during photosynthesis, during which carbon dioxide combines with water in the presence of radiant energy and enzymes associated with chlorophyll, to form glucose and release oxygen. The largest proportion of the biomass represented by green plants consists of carbohydrates such as glucose
All organisms need energy for maintenance, growth and reproduction. This energy is obtained from the conversion of the potential energy stored as chemical energy in food to kinetic energy, or the energy of work. It should be obvious that the total amount of solar energy fixed in the form of chemical energy represented by the biomass of green plants sets the upper limit to biological production and activity.
Solar energy is not trapped uniformly over the earth’s surface nor is biomass produced uniformly. The most important determinants of productivity are the cli mate, including precipitation and the extent of incident solar radiation, soil structure and availability of plant nutrients, and the efficiency of a particular plant community in trapping the incident available solar energy. Production is generally defined as the amount of organic matter elaborated over a specified time period, whether or not it all survives to the end of that time period. Several points have a crucial bearing on the transfer of energy and materials throughout an ecosystem.
- During natural transformations of energy from one form to another form, some portion of the energy involved is liberated as heat. Organisms cannot generally use heat energy directly to do work so there is a loss of total energy available to a biological system at each transfer. For example, when an animal releases the potential energy contained in glucose some portion of the energy becomes available for growth and activity (work) and the rest is lost to the environment as heat. In doing work, energy is also transformed into heat.
- All organisms have maintenance, growth and reproduction requirements. Energy is made available for these purposes by breaking down large food molecules into smaller molecules which are then further degraded to pro duce energy plus waste products in the process called respiration. Part of the energy produced during respiration is immediately lost as heat, the rest of the energy made available is used. First to maintain the organism; then to synthesize new biomass as growth or in reproduction , and finally as mechanical energy available to perform the various functions of the organisms.
- The producer trophic level made up of green plants has a very different chemical composition than the higher trophic levels. In particular, a given quantity of plant biomass has a much lower energy (caloric) content than the same quantity of animal biomass . A great deal of plant biomass must be degraded to turn the energy into the concentrated form found in animals.
- Finally at each trophic level the production is either passed on as food to the next highest trophic level, or it is passed on to the decomposers. Thus the herbivore food chain is continually releasing larger amounts of energy to the decomposer food chain.
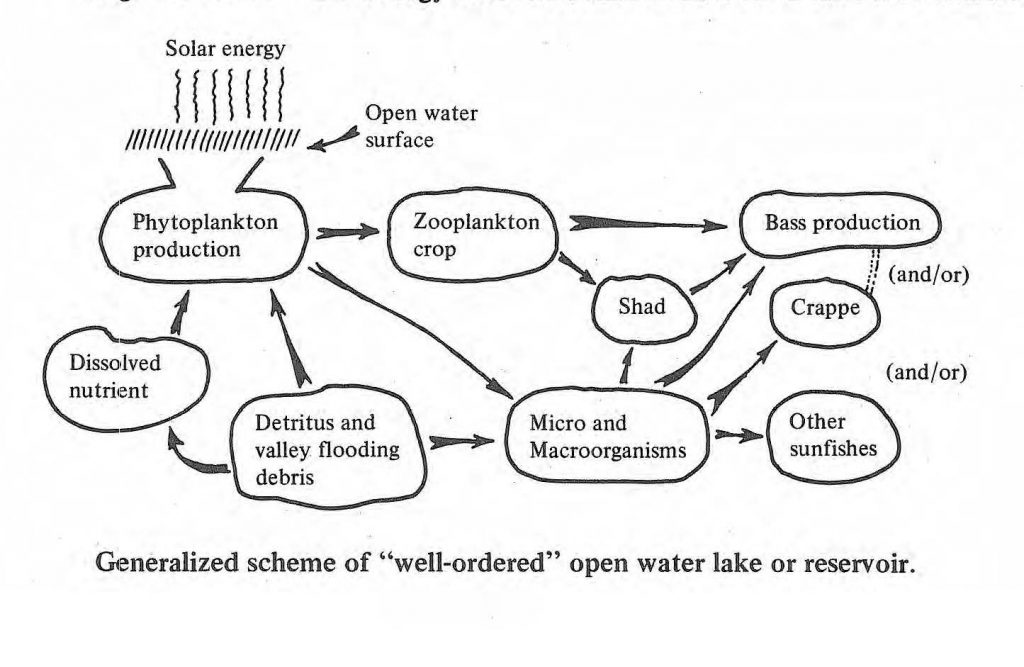
Open lake or reservoir
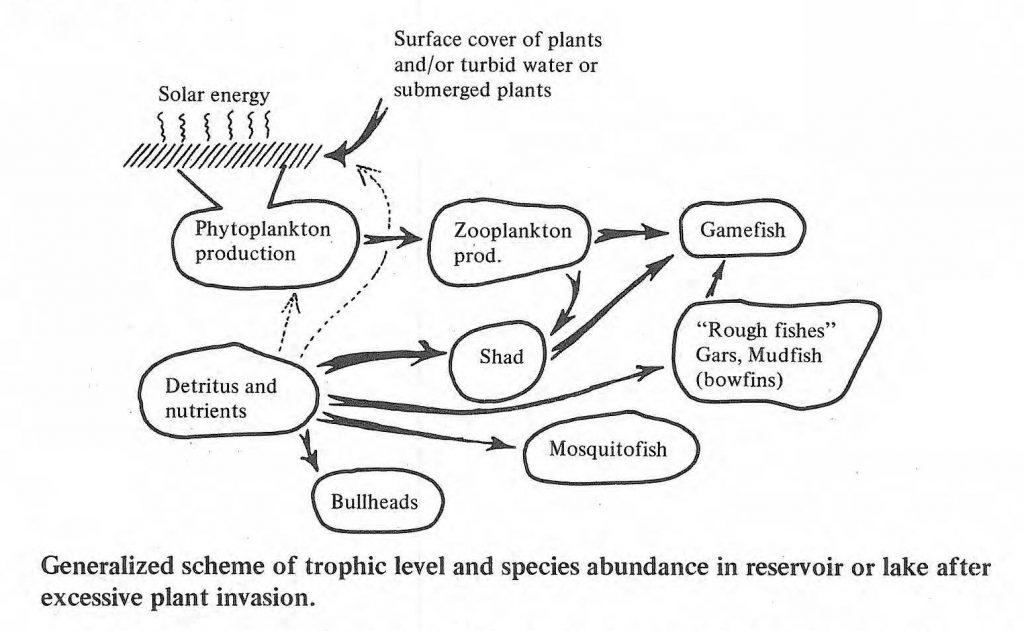
Lake or reservoir after plant invasion
A number of general conclusions can be drawn from this basic information. The amount of energy available to any trophic level over a period of time must decrease as we move up trophic levels. The maximum transfer rates being fixed at low levels by thermodynamic and biochemical constraints. The production of biomass must therefore decrease at each higher trophic level. It also appears that the efficiency with which food is converted into biomass is lower for herbivores than for carnivores.
Generally accepted studies indicate the existence of a pyramid of production of new biomass over a period of time.
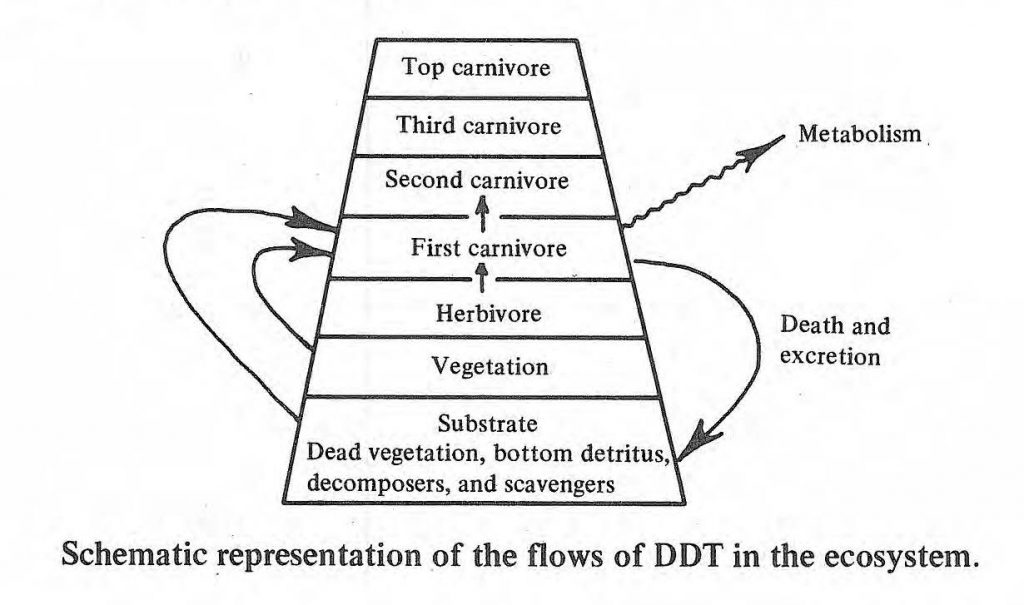
Trophic Pyramid; DDT flows
By contrast with the herbivore chain, over long periods of time the decomposer food chain consumes all of the incoming production and completes the dissipation of biologically fixed energy in the flow of heat.
The detritus food chain is of crucial importance in nutrient cycling and the same thermodynamic and biochemical principles apply as in the rest of the ecosystem. A system of trophic levels can be outlined, although perhaps less clearly. The individual organisms and the species involved in the detritus food chain are small and inconspicuous by habit, however, tremendous complexity exists in the system.
There are two distinct aspects of the decomposition process and both are required for completion. In the first, large pieces of detritus (dead animals, trees, leaves, and the like) are fragmented and partly decomposed, generally by invertebrates; then these coarse organic particles are decomposed into less complex inorganic compounds by simple organisms such as bacteria and fungi. The particular contribution of each organism involved in the decomposition process varies with the environmental parameters of the area in which the process occurs.
§4.4 Populations
A population is a group of organisms belonging to the same species and living in the same area so that there is a possibility of interactions among its members.
Populations are characterized by birth rates, death rates, age distribution, genetic composition and other observable characteristics. Under certain circumstances ecosystems are considered as being organized at the population level and interactions within and among populations are of concern.
The general academic discipline concerned with the organization of ecosystems by populations is called Population Biology, and that dealing with population abundance is Population Dynamics. ,
A population cannot be considered to the exclusion of other aspects of the ecosystem; rather it forms a focus and other component parts of the ecosystem are studied with a view towards how they influence the population.
The numbers of organisms in a population are determined by three processes- the birth rate (natality), the death rate (mortality) and movements.
Although the elements of population movement: emigration, dispersal, migration, are important in considering population growth and limitations upon growth, principal consideration has generally been given to birth and death rates. Populations increase in density when births exceed deaths and decrease when deaths exceed births. For populations which are approximately stable in numbers the birth rate must equal the death rate. Growing populations become limited in overall numbers either by an increase in the death rate or a decrease in the birth rate or some combination of both.
Since populations grow by multiplications, they have the capacity to increase in numbers at a constant rate. The numbers can double at any constant interval even though the numbers get larger at each doubling. This potential for exponential growth fortunately is rarely realized in nature for a variety of reasons.
There are a variety of important characteristics of natural populations.
- Self-regulation below the level of food destruction appears to be fairly common in some natural populations such as territorial birds and predatory animals.
- Changes in the quality of animals through time, by means of genetic change or physiologic change plays a role in determining abundance.
- In some systems, a species is more stable if it has a more complex or mixed age distribution.
- The history of a population is to some extent incorporated in its age distribution. The age distribution is a sort of population memory and the consequences of this history are carried into the future because of the existence of the age-distribution. This sort of demographic time-lag is well illustrated in certain human populations.
Populations do not increase indefinitely because all populations are constrained by limiting factors of some kind. Among the limiting factors and important considerations in population dynamics are the sources of mortality including enemies, competitors, food supply, available area, weather, internal population factors such as cannibalism, stress and waste build-u p. The factors affecting natality include food supply, opportunities for reproduction, nesting sites, weather, and interference among members of the population, such as territorial behavior in certain animal populations, stress and the operation of a social hierarchy. Stable populations are generally defined as those which are stabilized by density dependent process. Density dependent processes are those regulatory factors which increase in effectiveness as a function of increasing population density. Ecologists tend to look to such factors as food, enemies, nesting sites and interactions among organisms in the population for density dependent effects.
The separation of populations into stable and unstable is rather arbitrary. It is probably the case that there is a continuous variation from populations which are very stable to those which are extremely unstable.
The importance of the concepts of density dependence, population regulation and stability go beyond the realm of pure ecology into the area of environmental management. Although the problem of getting the optimum yield from an exploited natural population is complex, at its elementary level, for naturally stable stocks, exploitation should be density dependent over the long run to guarantee persistence of the population . Disregard of this obvious fact has led to the near extinction of certain species of whale. Similarly in the management of game-birds and deer, the aim is to kill the surplus each year by hunting and thus impose a density dependent mortality factor.
Many of the environmental problems of this generation are essentially population problems. Certain populations of small mammals noted for cyclic variations in numbers have been studied for decades and there are still several competing theories to explain the data. The reasons for the long times involved in population studies and their essential inconclusiveness reflect some of the unalterable characteristics of natural systems. Natural systems are complex and it is difficult to isolate the effects of interacting factors. Ecological systems, unlike physical systems are often non-Markovian, that is, the future state of the system cannot be predicted on the basis of the present state of the system but depends on the history of the system. Thus population events vary not only with locality, they are time-dependent as well.
§4.5 Communities and Ecosystems
Ecology can also be studied at the community level. Botanists in particular are interested in trying to describe communities in such a way that distinct assemblages of species can be categorized and classified together, while their limits can be defined and they can be separated from different communities. Plant Ecology was the earliest of the ecological disciplines and to many biologists, ecology is still essentially the study of plant communities.
It is often possible to recognize recurrent groupings of species which make up a large part of the community so that certain communities have come to have com mon descriptive designations generally representing the make up of the individual elements of the food web present in the area-the salt marsh, the deciduous wood land, the suburban community, the old field, the fresh water lake, the fresh water marsh or bog.
Another way of considering changes in communities is to study the changes as a function of time+succession. The succession of terrestrial communities under various circumstances has been fairly well described and occurs in an orderly straightforward way. Each stage or community tends to modify the environment making it more suitable for some other group of organisms, so that there is an interaction between the biological and physical elements of the system.
The production of plant biomass in early successional stages is high and the production/biomass ratio is high. As biomass accumulates and we move to a larger, older community with greater biomass, the relative rate of biomass production declines, the production/biomass ratio tends to decrease.
There is a trend during succession from an open to a more closed system with respect to nutrients. Climax communities tend to retain the nutrients they have and recycle them.
Ecosystems are linked by a flow of materials and energy and therefore are not truly independent of one another.
Fresh water bodies in particular are open systems. Thus streams gain nutrients in the run-off from surrounding terrestrial communities and there is a constant downstream drift of nutrients in the form of detritus and organisms.
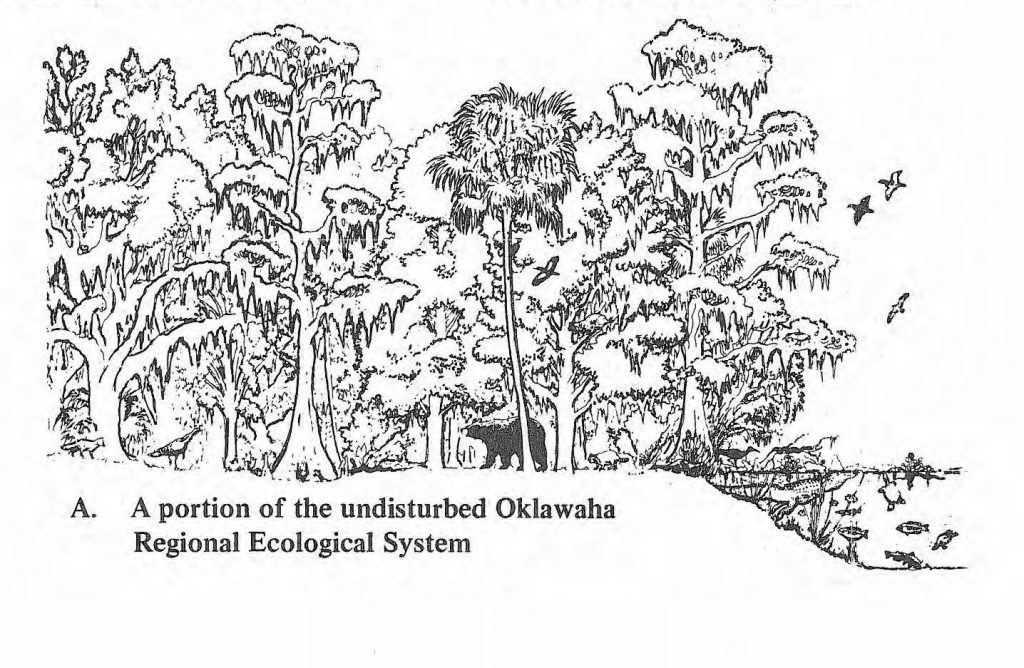
Undisturbed Ocklawaha Regional Ecosystem
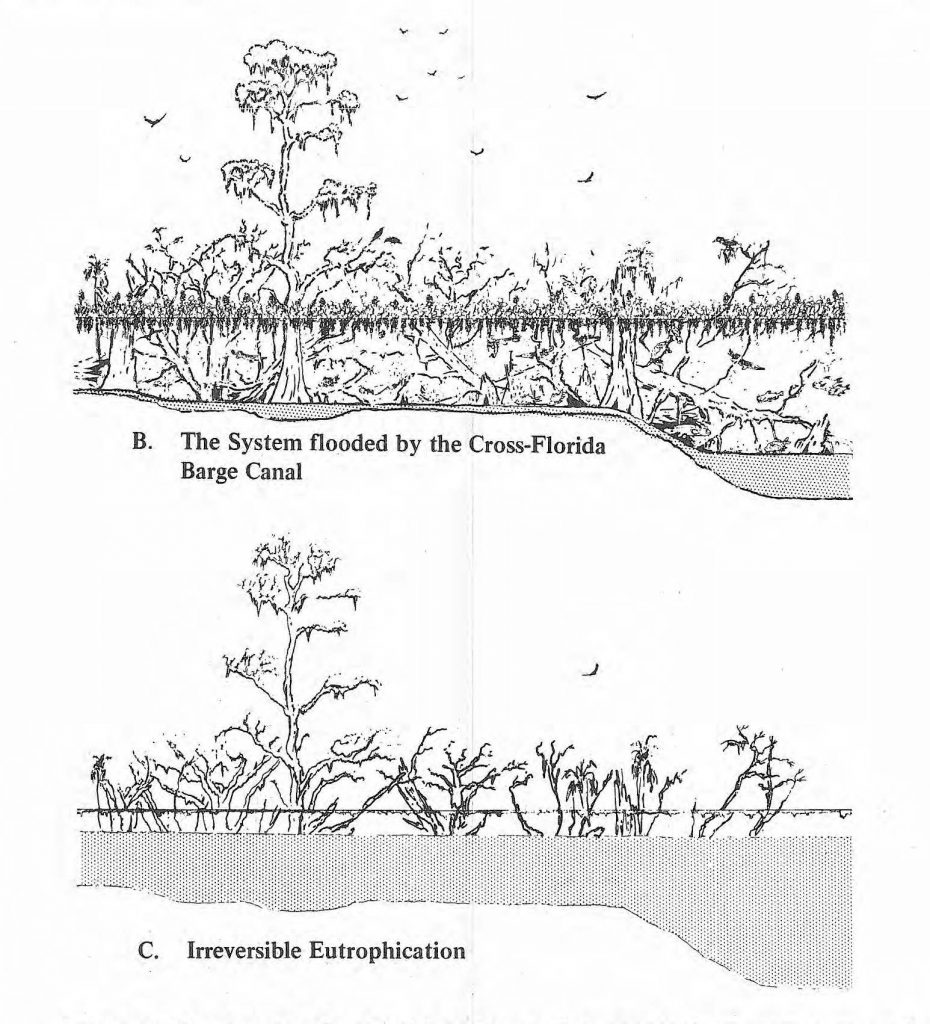
Ocklawaha system flooded by the Cross- Florida Barge Canal
In aquatic systems, however, not all of the material is passed downstream. In slow moving rivers and lakes, particles and dead organisms settle to the bottom where the organisms are decomposed, and the water body gradually fills up. A pond fills up and becomes a swamp during the process. As vegetation chokes the pond, the species of fish and other organisms change. The swamp is invaded by vegetation from the surrounding communities and terrestrial succession begins.
Ecosystems are dynamic, not only in that they are open systems, constantly receiving and losing materials, but because their character changes with time and they evolve into different kinds of systems.
The concept that different components of ecosystems interact, and that perturbation of one component of a system is likely to be felt elsewhere in the system, can be extended to interactions among different ecosystems, since all ecosystems are essentially open and liked together.
Migratory species illustrate the classic case of liked ecosystems. Populations of salmon, for instance, are greatly influenced by conditions in the freshwater river systems where they spawn.
The links between coastal marshes and ocean ecosystems play a great part in determining the productivity of the ocean. These _coastal wetlands are rich in nutrients and extremely productive. The young stages of many fish and shellfish feed there and then move out into more open waters. There is also a large movement of nutrients from marshes to coastal waters. Our seafood harvest depends upon the maintenance of such estuarine environments yet such areas are extremely vulnerable to development and also represent a sink for the accumulation, con centration and ultimate distribution of pollutants.
§4.6 Systems analysis and ecology
Systems analysis has become one of the most valuable tools available for the study of ecological systems.
Much of the material in this section is taken from portions of Systems Analysis in Ecology, edited by Kenneth E. F. Watt, Academic Press, N.Y., 1966; in particular Chapter 1, “The Nature of Systems Analysis,” by Dr. Watt, and Chapter 2, “Complexity of Ecological Systems and Problems in Their Study and Management,” by Dr. David Pimentel; Systems Analysis and Simulation in Ecology, 2 vols., edited by Bernard S. Patten, Academic Press, N.Y. 1971; amplified by personal communications with Dr. Watt, Dr. Orie Loucks, and others involved in the early applications of systems ecology to environmental law. Footnote attribution of sources is omitted, with thanks to all, however, the author takes responsibility for any errors or over simplifications which might appear.
Ecological systems are composed of many components which interact in a large variety of ways. Each biological component of the system is affected by the physical elements of the system, and all the variables change not only with respect to time, but from place to place since the environment is heterogeneous. Discrete components interact and each component of the system affects all the others in one way or another. The complexity of the system of interlocking cause-effect pathways con fronts us with a superficially baffling problem, and systems analysis is precisely designed to handle such situations.
In general, the system is analyzed in terms of its components. The processes affecting each component are analyzed and described so that changes with respect to time and distance can be described and ultimately predicted. The interrelationship of the components of the system is also analyzed and a model of the system is developed.
Eventually the model is tested by attempting to simulate, generally with the assistance of a computer, the consequences of alterations in the state variables representing components of the system.
In the case of a real ecological system, no attempt at simulation can be truly complete. Indeed, the art of systems ecology is to determine the crucial elements and processes that govern the general behavior of the ecological system as a system. Systems analysis is particularly useful to citizens and legislators who have to make decisions on less than a total data base.
Viewing an ecological system as an interlocking complex of processes characterized by many reciprocal cause-effect pathways, it can be seen that one of the principal attributes of a system is that it can only be understood by considering it as a whole.
Systems analysis has its roots in military and industrial operations research, applied mathematics, probability, statistics, computer science, engineering, econometrics and biometrics.
There are some standard approaches to dealing with the great complexities inherent in the considerations of real systems such as the operating maxim that com plex processes can be most easily dissected into a large number of relatively simple unit components, and that complex historical processes in which all variables change with time (evolve) can be dealt with most easily in terms of recurrence functions which express the state of a system at time t+1 as a function of the state of the system at time t. Thus the system is considered not in terms of its entire history but rather in terms of the cause-effect relationships that operate through a typical time interval. This idea of the recurrence relationship is common throughout mathematics. Matrices of transition probabilities in Markov processes are merely stochastic versions of a recurrence relation. Difference equations, differential difference equations, dynamic programming, and the “loops” of computer programs are all based on recurrence relations in which the output from each stage in the computation is the input for the following stage. No breakdown in this approach occurs if the state of the system at time t is a function of the state of the system not only at time t-1,
but also at time t+1, t+2 . . . t+n. This merely increases the number of variables
in the recurrence relationship and increases the dimensionality of the problem.
Another important basic principal of systems analysis is that optimization of processes is a central consideration. This premise brings to systems analysis the whole body of pure and applied mathematical theory related to the maximization and minimization of functions, the mathematics of extrema.
Combining the basic ideas of recurrence relations and optimization, leads to the concept that the purpose of systems analysis is to determine the optimal choice from among an array of alternative strategies at each of a sequence of times- the idea of the multistage decision process.
Multistage decision processes share two important basic similarities from a computational standpoint- high dimensionality and the need to be solved by some iterative process-requirements common to other types of problems that occur regularly in systems analysis: multiple linear regression, iterative, nonlinear regression, gradient methods for finding maxima and minima, and simulation studies in general. All problems leading directly to electronic computers for assistance.
Feedback control is another concept of systems analysis that is also important in ecological systems, so that a realistic mathematical description of a process includes terms such that deflection toward the equilibrium state, or steady state, follows departure from it within certain limits. Interaction, like other features of systems models is easier to describe in terms of changes at an instant in time rather than changes over a period of time, so that models of interactions are typically conceived of in terms of differential equations rather than algebraic equations. It is easier to consider a process in terms of the rates of change at an instant in time rather than in terms of the history of the process over a considerable period.
Inequality constraints are encountered commonly in ecological systems analysis problems, as are thresholds and limits. Similarly, the common technique of computer programming in terms of a cyclically repeated routine or “loop” is suitable for consideration of ecological problems where historical processes unfold through the repetition of variants of the same basic cycle of events, and dispersal occurs through a parallel process, but in space as well as time.
Another important concept from systems analysis useful in some ecological systems studies in that of information. The amount of information is related to the degree of order or negentropy (negative entropy) in a system and this concept plays a role in studies of community organization.
The principal reason for using systems analysis in ecology is the complexity of ecological systems. Ecological complexity originates from a variety of causes: number of variables; number of different types of variables (ecological, genetic, physical chemical, endogenous, exogenous); different levels of organization of ecological systems (populations, communities, trophic levels, cycles) and the nonhomogeneous and nonuniform distribution of system elements throughout time and space.
There is a definite sequence of steps required to properly investigate an ecological system for the purpose of describing its current condition or predicting its future course under different circumstances and the follow is an adaption of the method described by Dr. Kenneth E. F. Watt.
§4.6.1 Measurement
The first step in studying a complex system is to deter mine the variables and causal pathways that seem important in determining the function of the system. This list can be prepared from information in the literature, a priori considerations, casual field observations, or formal pilot studies followed by a scientific program of sampling the values of the relevant dependent and independent variables. The technical problems encountered at this step involve the techniques of measurement, sampling theory and application, logistics, and instrumentation.
§4.6.2 Monitoring
Finally, systems for continuous collection of some data over time are needed. In some cases basic elements for such data collection already exist and need only to be organized. Great quantities of social and economic data useful in pre-planning and determining the impacts of change are collected daily by units of local government. County registers of deeds, zoning commissions, county and municipal treasury offices and clerks, and many other agencies could be recording valuable information in coordinated form for automated data processing at a higher level of government. Such efforts could provide useful data at low cost for monitoring change and updating systems models. Other continuous monitoring efforts, such as studies of the effects of the release of specific industrial wastes into the environment, require specialized methods and technology, but such studies are essential in improving our ability to predict detrimental and costly effects in the future.
§4.6.3 Analysis
After all of the variables provisionally considered of importance have- been measured, it is necessary to evaluate their real relative importance. The field program will ultimately be revised to discontinue sampling those variables which do not significantly contribute to the 0verall definition of the behavior of the system, and only those variables which make a statistically significant contribution to the variance of the dependent variable need be included in the systems models. The ecologist concerned with analysis of a complex system is often led to the use of multiple regression techniques in conjunction with multiple analysis of variance. Additional complexities of interpretation arise if we use stepwise regression, or when certain variables enter the systems model non-linearly.
§4.6.4 Description
After it has been determined which variables need to be considered in order to fully describe the system, a model is structured. The first models are generally conceptual models which simply seek to fully describe the system and its behavior qualitatively without making any attempt to predict its behavior quantitatively.
A model is simply some method, usually a mathematical equation or set of equations, which can be used to describe the behavior of a system (a watershed, air mass, etc.). Complex mathematical models require the use of an electronic computer for solution of these equations. Model development includes comparison of predictions based upon the model with observed system behavior. If calculated behavior does not correspond closely enough to observed behavior, appropriate changes are incorporated in the model to make it more realistic.
A wide variety of models are available to describe the movement of water and substances contained in water, movement of materials in the atmosphere and the accumulation of substances in individual organisms or communities of organisms.
The importance of models is that they can often be used to predict the consequences of certain events or actions well in advance, thus allowing the public to consider positive and negative results before embarking on a costly and perhaps disastrous course of action.
The following list, derived from a report by the Faculty Land Use Problem Definition Seminar, “Data Needs and Data Manipulation,” available from the Institute for Environmental Studies, University of Wisconsin and authored by Lewis, Loucks, Moore and others, is intended to serve as an example of the specific data elements that appear to be necessary to provide an adequate description of environ mental systems and to provide a basis for planning and conflict-resolution are industrial siting, energy generation distribution and use. No attempt has been made here to determine scale, units of measure, or degree of specificity of the data. Such determinations can only be made in relation to specific problems and geographic areas.
A. Geological Data
l. Topographic maps
2. Soil family groupings
3. Soil reconnaissance maps
4. Soil associations maps
5. Land capable of supporting intensive farming
6. Engineering groupings of soils
7. Surface geology map
8. Bedrock geology map
9. Bedrock surface contour map
10. Precambrian surface map
11. Overburden thickness map
12. Glacial deposits map
13. Unique geological features: mounds, natural bridges, caves, sinkholes, waterfalls, fossil sites
14. Mineral resources maps
15. Active and abandoned quarries, mines, and pits
16. Aeromagnetic map
17. Seismic activity maps
18. Gravity map
B. Climate Data
19. Precipitation
20. Temperature
21. Mean relative humidity
22. Annual evaporation
23. Annual transpiration
24. Storm frequency and type
25. Wind velocity (monthly)
26. Prevailing wind rosettes (annual)
27. Atmospheric inversion probability map
28. Atmospheric particulates
29. Atmospheric sulfur
C. Hydrologic Data
Groundwater
30. Phreatic water surface maps (with observation well network delineated)
31. Potentiometric maps of major bed rock aquifer systems
32. Depth to water table (secondary map)
33. Quality- chemical (contoured TDS and/or separate maps of major cation and anion concentrations) near-surface (phreatic)
34. Quality- chemical (contoured TDS and/or separate maps of major cation and anion concentrations)- bedrock system (s)
35. Quality-particulates, in near-surface water
36. Quality- particulates, in bedrock water
37. Map of estimated total water storage volume from average-annual water table position to Precambrian surface
38. Groundwater recharge regions
39. Groundwater discharge regions
40. Map of estimated volumes of discharge
Surface water
41. Lake inventory maps
42. Flowing water inventory maps
43. Lake volumes (acre feet)
44. Stream storage: mean low flow
45. Stream storage: mean annual flow
46. Stream storage: maximum flow at flood state
Lake Characteristics
47. Miles shoreline
48. Existing reservoirs
49. Potential reservoir sites (based on topographic, geological, and hydrologic considerations)
50. Quality -particulates (mg/1)
51. Quality -chemical (by ppm of TDS)
52. Quality -chemical (pH)
53. Quality -chemical (specific conductance)
54. Sedimentation rates
55. Major point pollution sources by type
56. Mean annual lake level fluctuation
57. Temperature of hypolimnion
Stream/river characteristics
58. Quality-particulates (mg/I)
59. Quality-chemical (by ppm of TDS)
60. Quality-chemical (pH)
61. Quality-chemical (specific conductance)
62. Existing wild rivers
63. Wild rivers potential
64. Maximum depth
65. Bottom Characteristics
D. Biological data
Terrestrial elements
66. Native vegetation maps (including forest)
67. Native plant community types
68. Forest inventory maps (timber types by species, density, and age class)
69. Cultural vegetation type maps (man-induced)
70. Animal community types (insects and mammals)
Aquatic elements
71. Wetlands inventory types (including emergent aquatic plants)
72. Submerged aquatic macrophyte communities
73. Fertility and productivity (stage of eutrophication)
74. Aquatic invertebrate communities
75. Fishery resource types
E. Social data
(historical data should be assembled whenever appropriate)
76. Land ownership
77. Owner activity
78. Special interest areas or uses
79. Existing intensive land use maps
80. Population density
81. Population composition
Additional information recommended for storage and periodic updating, includes data with practical value as indices of land use trends.
82. land value trends
83. land speculation trends
84. public facility planning
85. semi-public facility planning
4.6.5 Simulation and Optimization
Once a model has been developed which accurately describes the behavior of a complex system, it can be used in simulation studies to show how the system can be managed in real life for optimal benefit.
A review of the preceding sections should suggest that the concern with accurate and efficient sampling, complex analysis of variance, regression problems and simulation studies, with statistical tests of the various models is leading ecologists into complex areas of mathematics. Model-building has led to an increased concern with differential and difference equations, partial differential equations and related topics of mathematical analysis. The concern with optimization and multistage decision processes has brought an operations research viewpoint into ecology leading to a concern with the literature in engineering and applied mathematics on gradient techniques, systematic search procedures and dynamic programming.
The sophisticated techniques of modern engineering have become a part of field ecology through telemetry, remote-sensing, satellite GPS, and the more frequent use of mobile telecommunications.
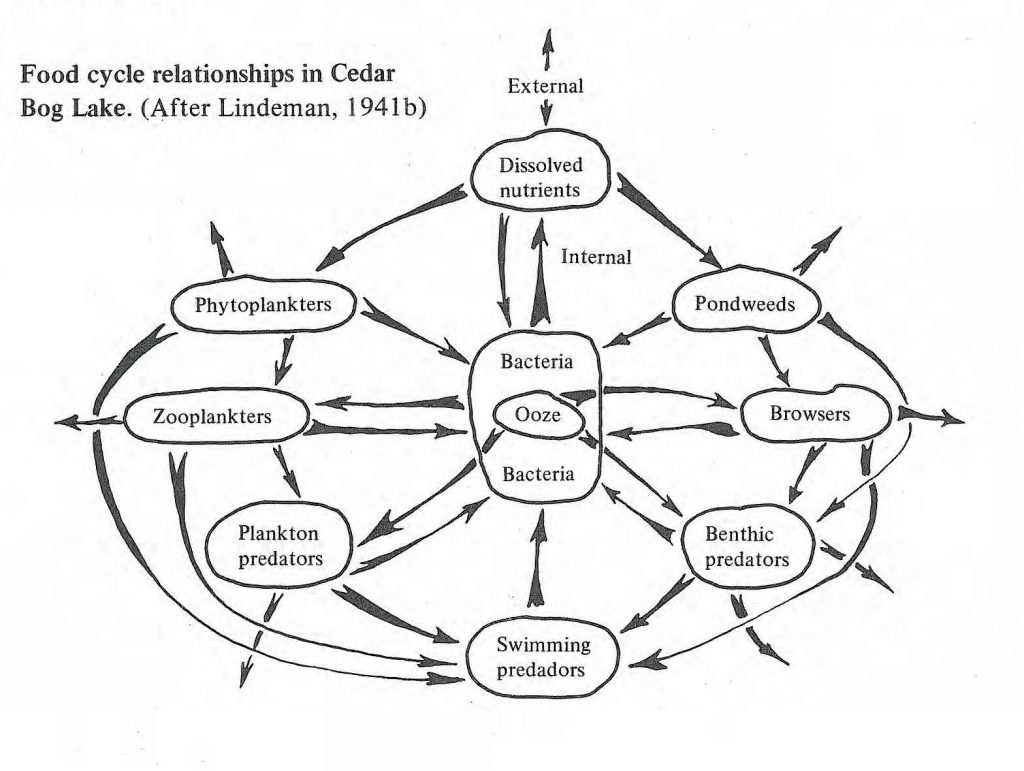
Food cycle relationships in Cedar Bog Lake
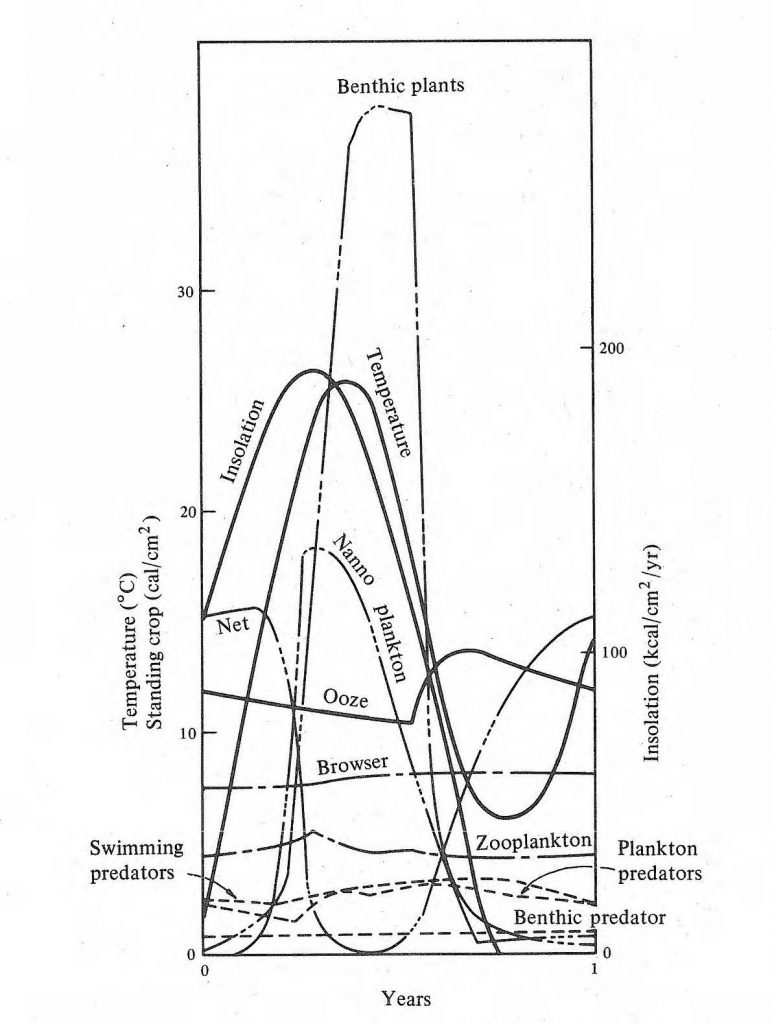
Behavior of final Cedar Bog model
§4.7 Impact on the Physical Environment
The effects of an industrial plant on its physical surroundings may include physical changes to the plant site, diversion of local resources, and changes in the off-site environment resulting from releases of materials, noise, and heat. There may also be indirect impacts from other development activities stimulated by industry.
The physical and biological portions of an ecosystem are so closely interdependent that they are often difficult to separate.
The severity of environmental impacts often vary widely, may often be reduced by appropriate changes in industrial practices.
While many industrial waste materials are foreign to natural systems, many others are normal constituents of the natural environment but may become “pollutants” if released in quantities sufficient to cause system changes considered detrimental. Even such common environmental components as water vapor or heat may be considered pollutants if introduced into the atmosphere or waterways in quantities sufficient to induce fog or icing conditions or to disrupt the fish and plant com munities of a stream by raising water temperature.
§4.8 Environmental Toxicants
The environment is now contaminated with a myriad of potentially toxic substances, many of which have now become constituents of nearly everything that man uses. In trace amounts certain substances are essential to life; yet in larger quantities these same substances may be toxic. The balance between these two levels is often unknown. Because of man’s own activities, substances not formerly present are now found in the human body. Many new substances are being formulated and new commercial applications for well-known substances are being found almost daily.
While approximately 2 million chemical substances are already known and several thousand new chemicals are discovered each year, most of these compounds are laboratory curiosities that will never be produced commercially. However, several hundred of these new chemicals are introduced into commercial use annually. Of particular concern because of their rapidly increasing use are the metals, metallic compounds and synthetic organic compounds.
Toxic substances enter the environment through complex and interrelated pathways. Manufacture, consumption and disposal are among the key processes for which human beings themselves are responsible.
Raw materials are extracted from the environment in a crude or natural form and then successively refined, processed and manufactured into more diverse and complex forms. The processes associated with manufacture may produce wastes to which man may be exposed at each intermediate step? Such wastes often contain not only the original substance but also modified, and perhaps more toxic, substances. The end products of manufacture are consumed by human beings as food or are used as durable and non-durable goods. Such consumption and use can result in further exposure to toxic substances.
Consumption, however, is not the end of most consumer products, because after they have served their often-limited purpose, they must be disposed of. Except for direct recycling, many current disposal methods return material to the environment, but almost always to a different place and often in a different chemical form. The disposal process alters the patterns of distribution and concentration of substances which naturally occur in the environment and may involve new chemical substances which may be more toxic than the original substance. Disposal processes often facilitate assimilation by living organisms, interaction with other chemicals and enhancement of inherent toxicity (synergism).
Both metals and synthetic organic chemicals are potential environmental toxicants, however, significant differences exist in the ways in which the two classes of materials enter the environment and ultimately affect the human species.
Metals, unlike synthetic organic compounds, have always been present in the environment, and living organisms, including man, have evolved in their presence. Blood and other tissues are composed of a complex mixture of chemical elements including the metals. Some metals are essential to life at low concentrations but are toxic at higher concentrations. In addition the form in which the metal occurs whether as a pure metallic element, an inorganic metallic compound or an organic metallic compound -strongly influences its toxicity. The danger to mankind from metals in the environment depends on the chemical form of the metal and its concentration.
Most synthetic organic substances are not essential to life, though many share with metals the characteristic of toxicity . As with metals, the concentration and type of exposure to a particular synthetic organic substance are key factors in determining its effects.
The total effect of all toxic substances on a single species, even humans, is difficult to quantify with accuracy because of the lack of knowledge about the sub-lethal effects of toxic substances. Although many environmental toxicants can cause death or injury in sufficiently high concentrations, the effects of long-term exposure to such toxicants at low levels, singly or in combination, are generally little known.
There are many difficulties inherent in the testing of chemical compounds for adverse effects. Extrapolation of data on dose effects obtained from animal studies to human beings must consider species variations in response. Toxic substances rarely occur in the environment in isolation, so that possible synergism or antagonism of two or more substances adds to the difficulty of adequate testing in the laboratory and interpretation of field data. Carcinogenesis, mutagenesis, and teratogenesis are often recognized as the sole significant biological effects.
Carcinogenesis is the ability of a substance to cause cancer. Chemical mutagenesis is the induction of genetic mutations- permanent and transmissible changes in the genes of an offspring from those of the parents of earlier generations.
Teratogenesis is the production of physical or biochemical defects in an offspring during gestation; it is limited to a particular offspring. The many deformed infants born of women who had ingested the drug thalidomide during pregnancy furnish a particularly horrifying recent example of teratogenesis.
Further complicating matters is the selective resistance of individual members of particular species. The effects of any given substance may vary among individuals of a single species as well as among different species. Differences in effects are often functions of age, sex, health condition and history, stress, different metabolic pat terns and other less understood factors.
After a contaminant enters the environment, it may be diluted or concentrated by physical forces, or may undergo chemical changes including combination with other chemicals that affect its toxicity. A contaminant may be picked up by a living organism which may further change and either store or eliminate it.
While the results of the interaction between living organisms and chemical substances are often unpredictable, such interactions may produce materials that are. more dangerous than the initial contaminant. A now classic example is the in organic mercury which was thought to settle safely to the bottom sediments when discharged into water. Now it is known that certain bacteria convert inorganic mercury into toxic, soluble, organic mercury compounds, such as methyl mercury, which pass throughout food webs eventually reaching man.
DDT, a synthetic organic chemical, is nearly insoluble in water, yet it is found in high concentrations among some fish-eating birds as a result of two factors: DDT is much more soluble in lipids (animal and plant fatty materials) than water so it selectively transfers from water to living organisms such as phytoplankton (microscopic plants that form the base of most aquatic food chains), zooplankton (microscopic animals that generally “graze” on phytoplankton), and this concentration process is enhanced by the process of biological or trophic level magnification, whereby successively higher concentrations of the toxicant are passed along toward the top of each food chain.
Synergism is another complicating phenomenon. Two or more compounds acting together or successively on an organism may affect that organism in a way greater than the sum of the separate effects of such compounds on the organism.
For example, the toxic effects of mercuric salts are accentuated by the presence of trace amounts of copper, cadmium acts as a synergist with zinc and cyanide in the aquatic environment to increase toxicity. Conversely, sometimes the presence of one substance lessens the effect of another substance of an organism. Arsenic, a toxic substance itself, counteracts the toxicity of selenium and has been added to poultry and cattle feed in areas where animal feeds are naturally high in selenium.
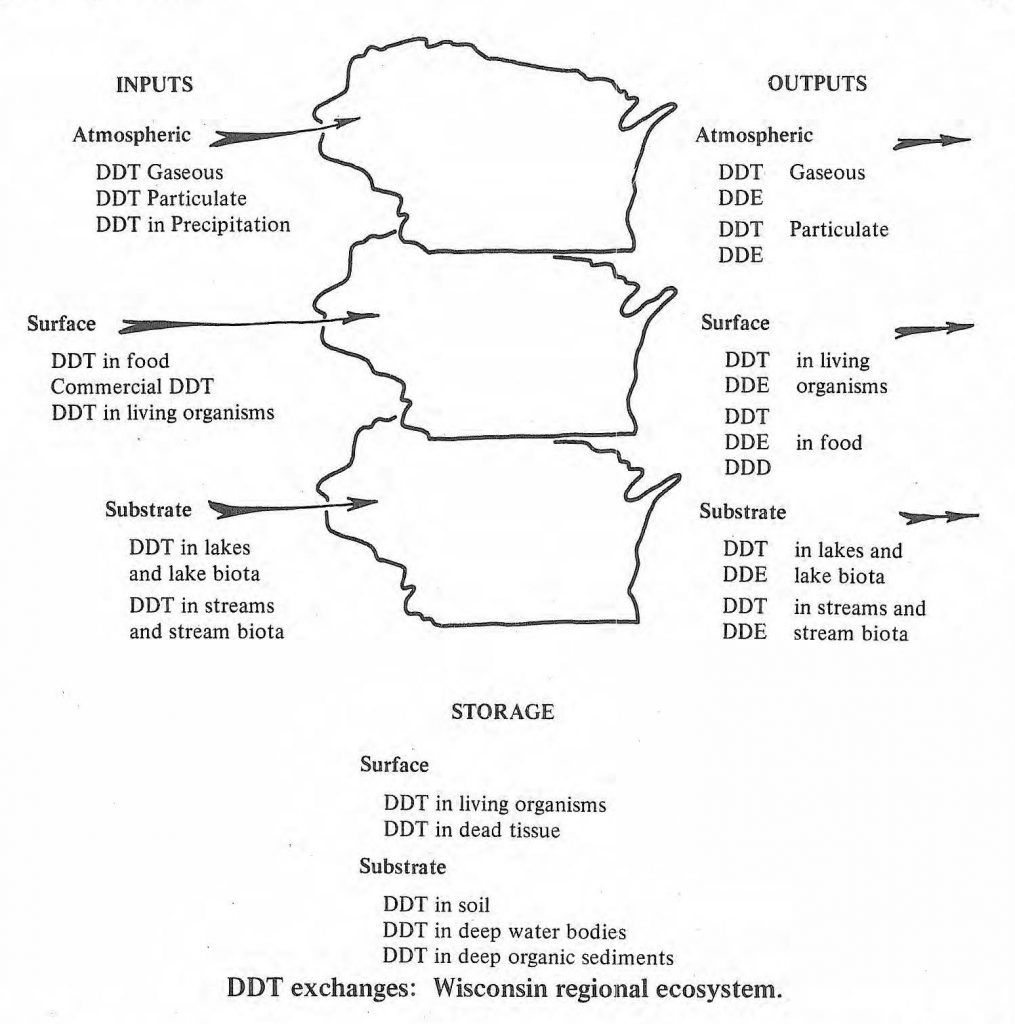
DDT exchanges
An additional problem arises because many environmental toxicants are not exclusively air and water pollutants but can be found in varying quantities at various times in air, water, soil, food and industrial, commercial and consumer pro ducts. The multiplicity of ways by which man can be exposed to these substances makes it difficult for agencies administering particular pollution laws to consider the total exposure of an individual to a given toxicant, a consideration essential for the establishment of adequate environmental standards. Also, no agency has considered itself completely responsible for all environmental toxicants present in and cycled throughout ecological systems.
In 1973, The agency most concerned with the total environmental distribution of its principle pollutants has been the Atomic Energy Commission where much of the significant work on material cycling and biological magnification has been done.
§4.8.1. Environmental Material Cycling
Once released into an ecological system, materials may be transported by the atmospheric circulation and by water movement. They may in turn be absorbed and transported by living organisms . Chemical changes may also give the substance properties quite different from those of the form in which it was released . Thus, inorganic mercury released into water ways, once thought to remain in lake and river sediments as an environmentally inert substance, is transformed biologically into methyl mercury . This much more toxic form is found in fish flesh and other organisms in water bodies receiving in organic mercury discharges. This physical, biological and chemical movement and transformation is often referred to as biogeochemical cycling.
§4.8.2. Geographic Extent of Impact
While the effects of waste releases are often local, atmospheric and hydrologic transport permit effects far from the source of the waste material. An example of this phenomenon is atmospheric transport of sulfur and nitrogen oxides. High acid levels are being observed in rainfall in areas far from industrial centers. The high acidity of the rainfall is attributed to the transformation of sulfur and nitrogen oxides into sulfuric and nitric acid. Sulfur dioxide originating in England and in the Ruhr Valley may be transported an average of more than 620 miles before reaching Scandinavian soil in rainfall.
§4.8.3. Effects Over Time
Environmental impacts may be separated in time as well as space from the introduction of waste materials. The time lags involved vary widely and depend upon the properties of the substance and organisms involved. An example of long-time lags is provided by DDT. A modeling study of DDT movement in food chains indicates that some organisms in a food chain are not expected to attain their maximum DDT concentrations in response to a constant level of DDT application for approximately four times the average lifetime of the longest-lived organisms in the chain. The magnitude of the time delays involved here become apparent when one considers that some predatory birds have life spans in excess of 50 years. Another modeling study of DDT shows that even with complete cessation of DDT application in 1972, DDT and its principle environmental metabolite, DDE are expected to be present in human tissues in measurable concentrations 50 years later.
These time lags point to a clear need to consider the consequences of waste releases in advance, since the problems which arise may be with us for a long time.
§4.8.4. Biological Concentration of contaminants
Many systems are capable of concentrating certain substances. This may occur simply by accumulation, such as the gradual buildup of sludge deposits on a stream or lake bottom, or such con centration may involve biological processes. Selective uptake and retention by organisms is a particularly important concentration mechanism. The degree of concentration varies widely and depends upon the organisms and substance involved, but some chemical elements have been found in aquatic plants and animals at concentrations up to 100,000 to 200,000 times higher than the concentration of the same element in the surrounding water. The effects of this concentration may be far reaching. Examples are the concentration of DDT and its metabolic breakdown products in certain food chains, and the concentration of mercury in fish food chains.
§4.9 Direct Environmental Impacts
Direct environmental impacts will be considered here as those effects upon the physical environment resulting from the actual generation and distribution of electricity rather than from development or other human activity stimulated by the power plant or transmission lines. Effects in water, air and soil will be considered separately.
§4.10 Water Related Impacts
Industrial activities may include both manipulation of local hydrology, such as use of groundwater and creation of reservoirs, and release of waste heat and materials to local waterways.
§4.10.1 Manipulation of Local Hydrology
Large scale pumping of ground water may result in both a lowering of the local groundwater table and a diversion of water needed elsewhere. Such effects are generally predictable using standard hydrologic techniques (non-equilibrium well hydraulic methods in conjunction with flow net analysis).
Construction of a dam generally raises the local groundwater table and may cause effects beyond the confines of the intended reservoir. Again, standard hydrologic techniques exist for the prediction of such effects.
Dam construction may significantly alter the quality of water released to the stream below the dam and may also reduce streamflow. Loss of water in reservoirs to evaporation is likely to be significant mainly in areas with warm climates. Water quality changes in reservoirs are often significant and may include a rise in water temperature and undesirable changes in the chemical content of the water.
Manipulations of hydrologic conditions may have effects beyond those in tended and these effects may often be predicted by use of standard hydrogeologic methods.
§4.10.2 Thermal effluent
Temperature is an environmental parameter of great importance to biological systems. Long term temperature shifts may cause pro found changes in natural community structure, and short-term temperature changes may radically affect the behavior or survival of individuals of many species. An ex tensive literature exists on the biological effects of heated water discharges.
A particularly large source of thermal discharges is the steam electric power generation industry. This source is becoming increasingly important with the trend toward construction of larger generating facilities and the increasingly frequent construction of nuclear facilities which release more heat into cooling water per unit of electrical energy than do fossil fuel burning plants.
The temperature change caused in a stream or lake by a given effluent release rate depends upon effluent temperature, the quantity of receiving water available for mixing with effluent, and mixing efficiency. The mixing efficiency is dependent upon the technique used in effluent release and the hydrodynamic properties of the receiving water body.
River flow rates often vary widely on a seasonal basis and the effluent may represent a considerable percentage of total flow during low flow periods, thus in creasing the temperature rise. Many hydrologic models exist for the prediction of water availability, and many models also exist for simulation of dispersion of thermal discharges in lakes and streams. The quality of predictions obtained from these models is variable, but many are sufficiently well developed that the range of thermal effects to be expected can be estimated.
Alternatives to thermal release directly into a stream or lake include the use of cooling towers, cooling ponds, or canals which allow some reduction in effluent temperature before release. Tradeoffs to be considered include the economic and aesthetic costs of large and relatively expensive cooling towers, meteorological and ecological factors related to the introduction of large amounts of water vapor into the atmosphere by some cooling towers which may cause fog and icing conditions, and the ecological, aesthetic and economic value of the relatively large amounts of land required by cooling ponds.
§4.10.4 Material Releases
Industrial wastes include a broad spectrum of subs trances too numerous to list here individually. Many of these compounds are known to be toxic, and the environmental and human toxicity of many others is unknown. Further complicating the picture is the fact that many wastes may be released by a large variety of industrial processes.
Materials released into aquatic systems may be either in dissolved or particulate form. Particulates will generally settle to the bottom, with larger particles lost close to the source and finer particles remaining in suspension longer, especially under turbulent conditions. Dissolved materials will usually be transported longer distances unless withdrawn from the water by organisms.
Suspended particulates generally decrease the aesthetic appeal of water, decrease light transmission, thereby reducing the amount of light available to vegetation for photosynthesis and may be directly detrimental to fish. Settling of particulates onto stream and lake bottoms may affect habitat suitability for desirable species of aquatic organisms.
Some organic compounds introduced into an aquatic system may be toxic, either directly or after concentration by food chains. A more common problem is depletion of the dissolved oxygen content of the water as the organic substances are broken down by oxidative reactions. Lowering of aquatic oxygen levels generally changes the species distribution of fish and other organisms present in a body of water, usually discriminating against game fish species. Periodic changes in dis solved oxygen content can result in large fish kills. The accuracy with which transport of materials by aquatic systems can be predicted is highly variable, de pending in large part upon knowledge of the environmental interactions of the substance involved and the complexity of the interactions. No generalizations can be made except to say that much success has been achieved already and that this is an area of active research. An example of such a model is the Hydrologic Transport Model developed in 1970 by Huff and Kruger, which has been successfully used to simulate movement of strontium-90 and cesium-137 originating in fallout from nuclear explosions through a watershed.
Models describing the effect of organic waste releases on dissolved oxygen content in rivers have long been in use. Mathematical techniques and data for prediction of movement of many substances through both aquatic and terrestrial food chains are also available. While data gaps exist and movement of all substances cannot be predicted with confidence, much is known about the hydrologic transport and food chain concentration of many substances.
§4.10.5 Impacts on Soils
Wastes released by industrial plants may eventually find their way to soils. In general, less attention has been given to the consequences of waste deposition on soils than to impacts on air and water.
One area of increasing public concern [in 1973] is the fate of potentially toxic materials deposited on soils. Some of these can accumulate in the soil to toxic levels, and some may be taken-up and concentrated by plants, thus finding their way into human food and animal feed. There are a number of unanswered questions regarding the pathways, retention, and toxicity of many elements in soils, plants and animals.
§4.10.6 Subsurface Geology
Groundwater hydrology is especially significant where extensive pumping of ground water is planned. Full knowledge of the characteristics of the aquifer is essential. The mapping of specific geologic formations is also critical in areas where seismic activity is probable. This consideration is especially important for installations in which rupture of containers would be disastrous, for example, power plants, whether nuclear or fossil-fueled.
§4.11 Indirect Impacts
Generating, distributing and utilizing electricity and siting an industrial plant may have physical environmental impacts other than those attributable directly to such activities. Some industries, in particular, electric power plants and high voltage transmission lines, encourage the location of other industries nearby. These industries may in turn cause environmental impacts such as those just discussed. The economic effects of the plant may also encourage local commercial and residential development.
§4.12 Impact on Biological Systems
This chapter considers the possible biological effects of generating, distributing and using electricity on the environment. It is sketchy but should serve as a quick guide to things the biologist knows that every concerned citizen should know.
A natural system is a complex interaction of plants and animals, air, water and soil and geology which maintains its existence as a system by using energy to cycle materials. Disturbing any of the parts of the system disturbs the entire system. Disturbing a system faster that it can absorb the disruption will destroy it.
The most commonly used method for evaluating environmental impact is a matrix such as that developed by Luna B. Leopold, Frank E. Clarke, Bruce B. Henshaw, and James R. Balsley of the United States Geological Survey and published as USGS Circular 645, Procedure for Evaluating Environmental Impact. It is the basic and essential essential tool for environmental impact assessment.
Obviously, any industrial development will change the natural system in its vicinity. It is the determination of which changes constitute harm that give trouble, since the dynamic equilibrium of the ecosystem is not only complicated and only partially understood but also subject to fluctuations, sometimes wide fluctuations. For instance, power plants are often built near water sources such as rivers and the flood plain of a river is a system which is subject to natural disturbance at regular intervals. This fact creates a necessity to assess changes wrought by human intervention against time. If a river floods and washes all the vegetation off a given area of river bank, there is a predictable succession of plants and animals that will return and grow there until the next disturbance, which may be years, or even centuries later. If that area is built upon, or otherwise appropriated by human beings and their works, the natural succession will not take place, and even though the building was put on what was empty land at the time, it has unquestionably altered the natural system. There are areas which are naturally disturbed by fire, like the chaparral areas of Southern California, which are changed by human suppression of fire. Equally real, although less obvious, changes occur all the time in any natural system.
§4.13 Assessment of Damage to Biological Systems
Ordinarily damage is calculated in human terms-monetary loss, or hazard to human health, however, these terms have broadened to include damage to (human) aesthetic or recreational values.
Even in human terms, harm is sometimes hard to assess if it is incremental and over a long-time span. Statistically, city dwellers get emphysema more often than country dwellers, but which air pollutant emitted by which industrial operation shortened which person’s life and by how much? Some sorts of environmental disruption have consequences which are not perceived for years. DDT, for example, concentrates in certain food chains and may demonstrate effects miles from the source and years after the application.
§4.13.1 Commercial and Game Species
Immediate losses of wildlife are one sort of harm arising from industrial development. If these losses include timber or game species, they are calculable in money, and are usually afforded legal notice. If industrial development is likely to injure a trout fishery, or a commercial oyster bed, or a stand of merchantable timber, courts have been convinced that such development entails harm to the environment. Commercially valuable species and game have received much study, and about them many trustworthy predictions can be made. There are governmental agency experts available to make such predictions.
It should be borne in mind that wildlife management techniques are directed toward increasing supplies of some species at the expense of others. What is the best thing to do for deer may not be the best thing to do for the forest, or the wolf, and, like any sort of human interference in a natural system, game management practices have far reaching consequences.
§4.13.2 Species Without Commercial Value
Species which have no value as game, food, timber or fur have, ordinarily, no immediately calculable monetary value. There are a few exceptions-species of extraordinary beauty or rarity which munity. The climax differs with the climate. One of the difficulties we see is an inability to measure harm to the community which would ordinarily come back the system not been altered.
§4.13.3 Loss of Diversity
For some years ecologists assumed intuitively that the complex interactions among species imparted to the community several characteristics that were more than those which could been attributable to the activities of each species taken separately. The whole seemed more than the sum of its parts. For the last seven or eight years the studies of diversity in ecosystems have legitimized this intuition. Diversity can be thought of as a web through which energy and materials flow and maintain the ecosystem. Intersections in the web represent species. Obviously the more species the system has the more options it has. A high number of options increases the probability that the system may recover if some part is damaged. The loss of a species may reduce the capability of the system to withstand sudden changes. The chestnut blight did not significantly affect the diverse forests of eastern North America, but the spruce budworm seriously reduced the amount of forest in the universe forests of Canada. This is the typical plant ecologist’s definition of diversity, simply the number of species pet number of individuals in the sample. An individual of a rare species has the same value as one of an abundant species.
However, among some animal ecologists and some plant ecologists, individuals members of rare species are thought to play more important roles than their more abundant neighbors. Evidence indicates that species may be rare because they use or convert rare materials under unusual conditions. If these materials are limiting, the dynamics of the whole ecosystem may depend on these rare species to an extent much greater than their numbers would indicate. These key species need not be spectacular to humans.
Several ecologists have suggested that diversity and thus stability increases geometrically with increase in contiguous area. Doubling the contiguous area quadruples the diversity. All else being equal the community might be considerably more harmed by taking a strip out of its middle rather than off one edge.
§4.13.4 Disruption of Natural Succession
Ecosystems are not fixed, they are constantly changing. If there is little human interference these changes are predictable. This predictable series of changes called succession ends in a climax plant community.
In southern states summer ambient water temperatures can be quite high in shallow waters. Shrimp, oysters and important fishes breed in the shallow, brackish lagoons along the intercoastal waterways. The young of these species are very sensitive to small changes in heat . A plant dumping its heated water into access channels can reduce hatching success and deprive the fish of large breeding areas.
These same brackish areas are very dependent on seasonal floods of fresh water from the rivers and streams that feed them. The young of many species can only survive if the water is sufficiently brackish, which requires the appropriate dilution of salt by fresh water during the spring. Fish, such as salmon, and shrimp have adapted their life histories to come in on the spring flood when the water is high and properly diluted. Many industries divert water or create impoundments upstream in order to store water in the spring for use during the dry season. This deprives the spring breeders of both enough water and water of sufficient dilution. Again as with heat, no one particular diversion may interfere with the spawning cycle, but the total of each incremental loss certainly does.
§4.14 Effects of Industrial Construction
An industry may affect an ecosystem either directly, as a result of any structures or paving or indirectly through its effluents. The construction itself displaces the plant community at the site.
The movement of air, water, and wildlife through an industrial site is usually disturbed . Any large building creates updraughts that affect migratory bird patterns, while millions of birds collide with towers, smokestacks and tall buildings each year. Residential buildings across the floor of San Bernadino Pass in Southern California have stopped the seasonal movement of desert animals through the pass. Dams, channels and intake systems have entrapped fish of a wide variety of species.
§4.15 Effects of Industrial Production
Industries have been known to introduce toxic materials and heat into air and water and to modify local weather. Toxic materials may be born in air or water. There are many reports of plants being killed by airborne sulfur oxides and ozone. Fish and aquatic plants have been killed by chemicals, particulate matter and organic material in water. The decaying organic material may produce materials toxic to other life, but the greater effect is that organic materials use up the oxygen needed by other organisms and deprives them of oxygen, nutrients and light.
Although toxicity of materials was historically defined in terms of human health, toxic effects on fish and wildlife now must be considered. In some cases toxic materials are hard to identify because of synergistic interactions. Even when the ma1terial is a well-known poison such as cyanide, the evidence linking the discharge of cyanide to a particular fish kill in the Houston Ship Channel was circumstantial. For environmental toxicants such as DDT and the PCB’s which are very long lived and widely used and which accumulate throughout biological food chains, individual responsibility is particularly difficult to establish.
§4.15.1 Heat
The introduction of heat into a lake or stream has varied results. In some cases it has been shown to improve a warm water game fishery, while being only weakly linked with early and severe algal blooms in others. In some areas, waste heat keeps parts of lakes open all winter attracting Mallard, Black Duck and Coot to overwinter. However, since there is scant winter forage, these birds must be fed. In some cases the heated water encourages fish to feed and be active, while at the same time killing the potential food supply. In Lake Michigan local thermal pat terns in the water may cause IS°F. changes in a few minutes, making measurements of the ambient temperature and the impact of effluent uncertain. When, as in the case of Lake Michigan, the amount of effluent is very small compared to the volume of the lake, the impact seems small, however, a substantial increase in thermal effluent, could materially affect the quality of the lake fishery. Here, as in many cases involving multiple use of public resources, no one use causes the ultimate degradation of the resource which really represents the sum of each incremental addition.
With the drying of the Everglades, the beautiful Everglades kite is threatened by the loss of its food supply. Many bird lovers are vigorously trying to protect the kite, but there doesn’t seem to be much concern for the passing of the snail that it eats. “Save the Everglades snail!” is just not an effective rallying cry.
The same is true of many species of small fish, insects, plants, and amphibians. No one knows “what they’re good for.” They are unlikely to have been studied intensively, and their ecological contributions are likely to be unknown.
There is also a possibility that industrial development, especially in un developed areas may harm or destroy species which are not even known to be pre sent, since many areas of this sort have never been assessed biologically.
§4.15.2 Weather
Local weather results from the interaction of moisture and heat, both of which may either originate locally or be brought in by moving air or water. Huge areas of concrete do not store water and tend to retain heat. As a result cities are usually warmer than the surrounding countryside. The increased heat further a5gravates the dryness and dustiness by increasing the evaporation rate. Small factories have an effect on local air currents. Summer thermal uprisings can be detected by watching soaring birds.
Moisture regimes are modified both by drying and by the addition of moisture via cooling towers and cooling impoundments. Desert plants (Saguaro) have been reported to die as a result of increased humidity caused by the large impoundments along the Colorado River.
§4.16 Irreparability of Biological Systems
Once the possibility of damage has been established , the next problem is to determine whether the harm is irreparable . Some sorts of biological losses are renewable. For instance, along the northwest coast selectively cut conifers will be replaced within a reasonable time. Some sorts of losses seem renewab le, but require new energy inputs into the system, such as tim ber replacement in a slower-growing forest which requires the addition of fertilizer. The substitution of faster growing trees for slower reduces diversity, like replacing a mixed southern hardwood forest with a slash pine plantation, so that measuring “renewal” in terms of board -feet of lumber may be misleading.
Other environmental impacts may reduce animal or plant species for a time, and then the question of renewability revolves around whether conditions have been so changed as to preclude restocking, and if conditions favor restocking, whether there is a sufficient population of the species elsewhere to provide the breeding stock.
If an industrial plant dumps a toxin into a stream once, and kills all the fish, then stops dumping the toxin, the fish will probably return. Unless an entire species is completely destroyed in the kill, in which case, the loss of that species would be irreparable. If the effluent permanently changes the character of the stream so as to make it uninhabitable by some species, the condition would also be essentially irreparable.
Changes, such as soil erosion, timber cutting, water pollution or air pollution, can make a relatively stable system, unstable; while other changes, such as fire and flood control and breakwaters, can artificially stabilize a relatively unstable. Either may constitute irreparable damage to the system as a whole.
§4.16.1 Cost of Repair
A number of industrial sites have planned and built natural areas as part of the landscaping around their buildings. Usually the dominant plant species are introduced, and often exotic species are used. These , plantings, while aesthetically pleasing and of educational value, do not immediately become the ecosystems after which they are modeled. They may come close after 20 years or so with grasslands and four times that long with forests. These small projects are in ideally very expensive, so that we can see that it would take considerable time and money to replace a natural community that cost so little to destroy. Replacement cost would be a useful method to determine the value of lost ecosystems. The same could be used for species, however, an extinct species is impossible to replace and therefore priceless or valueless depending on your point-of-view.
§4.16.2 Rate of Repair
Natural ecosystems vary in the rates at which they may be repaired. A trampled sand beach can be substantially restored on the next high tide; but a single footstep on alpine tundra can kill a forget-me-not the size of a quarter that has grown for 75 years. Chop down an aspen grove and it can return in 10-20 years, but climax redwood or high-altitude pines may take 500–1,000 years. If the land is plowed or graded, the pines and forget-me-nots will never come back even if left alone. The redwoods might recover in several hundred years and the aspens in 30–50. The rate of repair is an indication of sensitivity of the system and magnitude of harm done to it.
§4.17. Impact on Air
Air pollutants are conveniently divided into two classes: gases and particulates. Particulates are transported distances which depend on their size as well as meteorological conditions, with the largest particles being deposited closest to the source. Deposition processes include fallout, or simple earth ward movement by gravitational acceleration, washout, the sweeping of materials out of the atmosphere by falling precipitation—the formation of water droplets or ice crystals around particulates which serve as condensation nuclei, and subsequent are removed by precipitation.
Gaseous wastes are transported by the atmosphere until removed, usually after sorption onto water droplets or other particulates. Physical, chemical and photo chemical processes may transform such wastes during transport. An example is the chemical transformation of sulfur dioxide to sulfur trioxide, which may then combine with water to form sulfuric acid, resulting in acid rain.
Impact of atmospheric particulates ranges from soiling of surfaces to human illness arising from their inhalation. Atmospheric transport provides a mechanism for accumulation of toxic materials in surrounding ecosystems to levels at which they may become damaging.
Impacts of gaseous wastes range from human illness to corrosion of man-made structures.
Technology exists for removal of up to 99% of particulates from atmospheric discharges, (See §3.19.1), however removal of gases from atmospheric discharges is usually more difficult. ·
Movement of wastes discharged into the atmosphere depends upon the height of the stack used, local topography and meteorological conditions. A large variety of models exist for simulating atmospheric transport of materials, including those originating in single point sources, line sources, (such as a roadway), multiple point sources, and area sources. Multiple point source and area source models lend them selves well to urban situations. Area source models in particular lend themselves well to air quality management through land use regulation.
Model precision and accuracy varies depending upon the model used, input data available, and the type of prediction sought. In general, predictions of concentrations averaged over time and space are more reliable than predictions for a particular time or for a small area. Predictions for gas transport tend to be more reliable than those for particulates.
§4.18 Problems in Determining Causes of Environmental Damage
We have just reviewed the immediate environmental damage which might result from industrial impact. These are the most obvious effects to assign causes for, but they may not be the most important.
§4.18.1. Space
Some effects are separated from their cause by intervening space. For instance, an industry may require electricity, and result in further impact on the area where the electricity is generated. If water is impounded, not only is the area which is flooded affected, but the character of the ecosystem downstream may be radically altered. Smoke may change weather downwind. It is necessary to con sider a wide range of effects in order to fully understand the impact of generating, distributing and using electrical energy.
§4.18.2 Time
Other effects can be separated from their causes by time. Persis tent toxins may take years to concentrate in the food chain sufficiently to do measurable harm. Reduction of breeding space may endanger a species but not be apparent for as much as twenty years.
§4.18.3 Synergism
To complicate the problem still further, two or more causes can combine to result in an impact greater than either would have had alone.
§4.19 Social and Economic Effects of Industrial Development
Determination of the environmental impact of industrial plant siting must consider the effects of industry on the socio-economic environment of a region. The social and economic effects of industrial development vary widely with the type of industry and the characteristics of the region in which the industry is locating. This section delineates some of the regional characteristics which determine the extent of impact.
The social and economic effects of an industry locating in a suburb of a major metropolitan center will differ drastically from those of an industry locating in or near a small agriculturally oriented rural town.
§4.20 The Metropolitan Suburban Setting
The impact of an industrial plant in a metropolitan suburban setting is extremely complex. A metropolitan suburban community whose principle growth has been in population rather than economic activity (the residents work outside of the community) usually finds itself in a disadvantageous tax position. Extensive and costly systems of services and facilities have been provided from a property tax base which has a relatively low value per unit of land area (residences). When an industry with a relatively high value per unit of land area enters the community, the tax position improves and more development occurs. A suburban community with extensive facilities and services usually incurs far more benefits in tax dollars from new industry than the additional cost of facilities and services required. Favorable labor force characteristics, extensive public services and facilities, and the presence of new industry may induce related industries to locate in the community. More services are then needed to support the new industrial firms and their employees and more jobs are created. This continuing cycle is often called a multiplier effect.
The effects of an industrial plant siting in a metropolitan suburban community will very likely include associated or support industry, industrial and business services and increased retail and service businesses to support the growing work force. Sewage and water facilities, highways, and community services expanded to accommodate the new growth may be attractive to other industries totally unrelated to those already in the community. Residential areas will also expand to house the growing work force.
The relationship between the indirect social and economic effects (through land use) and the natural environmental systems becomes immediately apparent. Additional industries may increase existing pollution levels. All development dis places natural communities and may drastically alter the hydrologic system especially quantities and patterns of storm-water run-off.
Multiplier effects caused by industrial plant siting in a rural setting are not as great.
§4.20.1 Changes in age composition
Social demographers have long been aware of a surplus of old people in small towns and rural counties. As early as 1942 Smith labeled the small town as “America’s old folk’s home”. Two demographic trends: (1) the steady out-migration of young adults and (2) the in-migration of retired urbanites and agricultural people, have produced a top-heavy age distribution in these areas. This abnormal age distribution is an important economic determinant in many rural areas and small towns.
One of the major arguments for bringing industry to depressed areas is that the expanded employment opportunities will stifle the out-migration of young adults and thereby re-establish a more or less normal age structure, and research has generally substantiated this argument.
§4.20.2 Increase in Income
It is well known that many small towns and rural counties are lagging in terms of economic growth. One of the major reasons these communities are attempting to attract industry is to establish a stable economic base and thereby raise the general level of living of their citizens.
§4.20.3 Increased employment
Many residents of small towns and rural counties are underemployed. Advocates of industrial siting in these areas argue that the expanded employment opportunities will decrease unemployment and under employment, and, increase employment not only in jobs at the new plant but also result in a multiplier effect- generating employment in other areas, e.g. filling stations, restaurants, construction, and the service industries.
Income and employment changes take two forms-direct and indirect. The direct change is the income (payroll) and employment at the new industrial plant. The indirect change is the income and employment caused by the new jobs and new income at the plant. An example of indirect employment change is the hiring of more clerks by merchants to handle the increased business caused by the new plant and its employees.
The indirect impacts are commonly measured by a multiplier . The employment and income multipliers, while similar, differ in magnitude because the jobs generated in different sectors of the economy pay different wages. The multipliers can measure both direct and indirect change or indirect change only.
Several techniques exist for analyzing the economic and spatial relationships among industries. Inter-industry analyses are premised on the concept that the ties or linkages between businesses transmit changes in economic activity from one business to another. For example, an increase in steel production can lead to increased activity in the transportation industry increased coal production for coke, and additional activity for businesses servicing the iron mining industry. The export-base and inter-industry approaches both make comparable estimates of the indirect economic impacts. The inter-industry analysis provides a more detailed description of the changes, but this comes at the expense of increased data costs.
§4.21 Limitation of Analysis Techniques
While the techniques to estimate the multipliers are generally accepted, there are limitations to the techniques that must be considered and the results are not general. The multiplier for a manufacturing firm locating in northern Wisconsin can vary greatly from the multiplier for that firm if it located in metropolitan Chicago. The reason is that the necessary supporting and linked services for the firm are likely to be available locally in the Chicago area but not in northern Wisconsin. The indirect effects from the new plant still occur but they occur somewhere other than in northern Wisconsin.
The reverberations from a change caused by manufacturing can vary significantly from a change caused by wholesale and retail trade.
The geographic spill-overs are important considerations when evaluating economic and environmental impact of industrial development. But geographic spill overs are only recently being empirically examined by the techniques of export-base and inter-industry analysis.
Inter-industry analysis provides a clue to the need for supporting business services by an industry but fails to incorporate fully the spatial aspects, i.e., where the supporting firm locates. Another technique, industrial complex analysis, is an attempt to combine the spatial aspects with inter-industry analysis. This technique attempts to minimize the costs to a subsystem of activities (not a single industrial sector) which are subject to important production, marketing or other interrelations. While this technique is not designed for the prediction of ancillary businesses, it appears to be useful for that purpose.
§4.22 Negative Effects
While the positive social and economic effects of industrial development are fairly straightforward, the negative consequences of such siting have received much less attention. Several have argued that, since most Americans equate industrial development with progress, there has been little concern over the possible liabilities of such development. The severe social, economic and environmental problems that plague our over-industrialized metropolitan areas, however, clearly indicate that industrialization is not the panacea for all problems. Some of the most important potential problems of locating large industry in small towns are:
- income inequility,
- “leakage” of benefits, and
- size and density of population.
§4.22.1 Income Inequality
Although industrial development generates an in crease in per capita income these increases are not proportionately distributed throughout the population. more specifically, some very sizable segments of the population may actually be adversely affected by industrial development.
Older people comprise a large portion of the population of small towns and rural counties, and many of these elderly individuals settle in such areas to take advantage of the fact that the cost of living is lower than that of large cities. When a large industry moves into the area, however, the entire economic structure of the region is altered. Older people, already at the lower levels of the economic hierarchy, are likely to be relegated to an even lower economic status as the income of younger individuals sharply increases.
While the absolute income of the elderly may not decline, their economic status relative to younger residents of the area can be drastically reduced. Given the fact that the elderly people are already concentrated in small towns and rural counties this problem should be recognized by those who would encourage the industrial development of these areas.
A second segment of the population which may be adversely affected by industry consists of the female heads of households in the area. Many of these female-headed families are located in small towns and rural counties which are actively seeking industry. And the type of industry which communities are most eager to attract are heavy manufacturing facilities. These plants have predominantly male payrolls. Thus femaie household heads in the area may find themselves in the same position as the elderly—unable to take advantage of expanded employment opportunities. While not all industries have such a high proportion of males on their payroll it should be re-emphasized that the types of industries which most communities are seeking to attract have a high male specific labor demand. (Note: This was written in 1973. Not much has changed for women in the workforce since then, unfoprtunately. While some barriers to employment of women have fallen, equal pay for equal work is still not accepted throughout the labor market.)
§4.22.2 “Leakage” of Benefits to Surrounding Areas
Large commuter fields often develop when a plant is located in a rural area or a small town. In many instances people commute to the plant from more than 50 miles away. This indicates that the benefits of the plant may be spread over such a wide geographical area as to have a dilute effect. While this phenomenon may not at first appear to be a problem it can easily become one if leakage exists to such an extent that the community in which the plant is located receives only a small share of the benefits but almost all the burdens of the new industry.
Many communities, eager to attract industry, will develop industrial parks, provide tax subsidies, expand the school system, and revamp the transportation system, all with the idea of recouping these expenses somehow when industry locates in the community. But when the siting actually takes place the benefits may be distributed over such a wide area that the community cannot regain its expenses let alone attain economic stability.